Interlocking – Production-induced properties in interlocking of lamination stacks for stator cores
To reduce iron losses, the soft-magnetic rotor and stator cores of electric drives are built up from thin, electrically insulated individual laminations. In addition to the properties of the semi-finished products and the sheet thickness, the mechanical and electromagnetic properties of the motor are influenced by the process for joining the laminations. One manufacturing process that represents an economical alternative, especially for large quantities, is interlocking.
In this project, a comprehensive examination of the influences of component design, tool and process parameters during interlocking is to be carried out and a model for the joint strength derived. In addition, the basis for real-time process monitoring by means of an AI-supported analysis of process variables is to be established. The findings are to be recorded in the form of a recommendation for action.
Motivation
Due to increasingly stringent requirements for technologies with regard to reducing greenhouse gas emissions, the demand for energy-efficient electric motors is rising. In the transport sector, an acceleration of electrification is also supported by political measures, leading to an increasing amount of electrically powered vehicles among new car registrations. In order for electric drives to compete technologically with fossil fuels, vehicle manufacturers are striving to increase ranges while reducing the weight and installation space for the drive and energy storage systems. One step in this direction is to increase the efficiency and power density of electric motors, resulting in a reduction in overall weight.
The rotor and stator cores, which are usually made of soft-magnetic materials, i.e. materials with low coercivity and high magnetic permeability, offer particular potential for optimizing electric drives. Iron-silicon and cobalt-iron alloys, which are characterized by high saturation induction and magnetizability, are used for this purpose, which is important for achieving high torque densities.
The iron cores of rotors and stators are generally not solid but made of individual laminations which are electrically insulated from each another and are designed to be as thin as possible to reduce eddy current losses.
Various processes exist for joining the individual laminations into a laminated core. One of these is interlocking. Following the forming process (usually a shear cutting process), this is subdivided into the stamping and stacking process steps (see Fig. 1 top). The principal mechanism of interlocking is based on an interference fit. The bond strength is created by pressing the previously embossed spots into each other by applying axial force. Sheet thicknesses in the range of 0.30 mm to 0.35 mm (and in some cases even less) have been common practice for interlocking processes for both NO electrical steel and CoFe.
The process is extremely complex due to a large number of manipulated variables (cutting clearance, stamping depth, counter punch force etc.) and disturbance variables (e.g. wear and semi-finished product fluctuations) as well as narrow tolerance ranges, so that the relationships between the process parameters and the resulting magneto-mechanical properties have not yet been sufficiently investigated. For this reason, the process design of interlocking is based primarily on empirical knowledge.
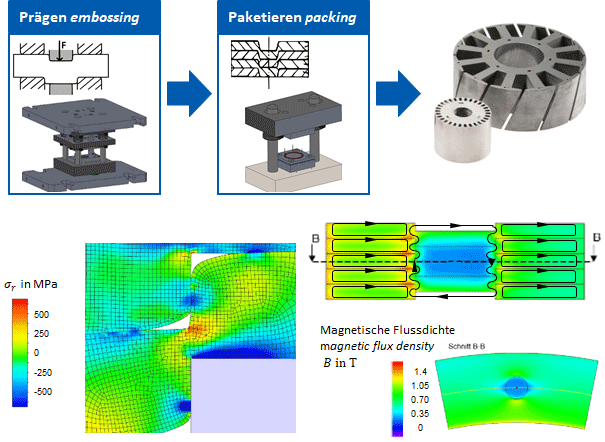
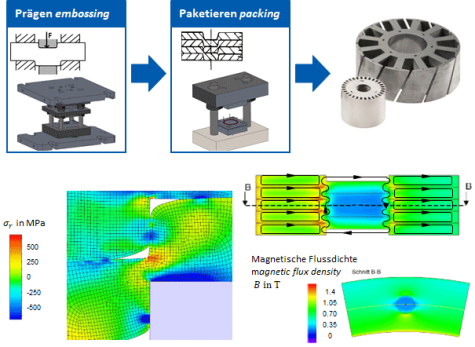
Approach
Currently, an insufficient data basis on the process limits in the interlocking of components for electrical energy converters restricts productivity. Consideration of manufacturing-induced properties in product and process design can lead to optimization of mechanical and magnetic properties. In addition, by monitoring process variables, real-time statements can be made about the condition of the interlocking spots, which enables resilient process control and thus an increase in quality and productivity. The following overriding objectives are derived from this for the research project:
• Development of know-how with regard to the design of interlocking tools and the mechanically packaged connection (spot design)
• Optimization of the process chain through possible elimination of heat treatment with precise knowledge of the stress state after the joining process, as well as through resilient process control based on monitoring of in-process variables
• Optimization of electrical energy converters by considering manufacturing-induced properties in product design.
Therefore, two questions in particular are addressed:
1. How do manufacturing-induced properties during interlocking of rotor/stator laminations affect the magneto-mechanical properties of electrical components?
2. How can these properties be specifically influenced?
To answer these questions, a model-based description of the mechanical and magnetic properties of interlocked components is necessary (see Fig. 2 bottom). The models to be developed during the project for describing the magnetic and mechanical properties of stamped-packaged sheet packages based on of finite element calculations will be validated using experimental data. To this end, standardized test ring packages will first be analyzed. With the aid of the validated FE models, parameter studies are then carried out using a real stator geometry. The findings obtained in this way are in turn validated with experiments.
In this way, relationships can be derived between the joint strength, the process boundary conditions (tool setting, stroke speeds, material etc.) and the magnetic properties of the sheet packs (eddy current losses). As a central step, a model is to be developed for describing the mechanical joint strength of embossed spots during stacking. By recording force-displacement signals during the process in combination with AI-supported analysis, it will ultimately be possible to identify faulty process states and predict the quality of the joint. Machine learning approaches must first be used to transform the recorded time signals and derive characteristic data. Finally, regression models for predicting the joint strength are developed on the basis of these characteristic values.
Acknowledgement
The research work presented here takes place within the framework of IGF project no. 22036 N of the European Research Association for Sheet Metal Working (EFB). This is funded by the German Federal Ministry for Economic Affairs and Climate Action (BMWK) via the AiF as part of the program for the promotion of joint industrial research (IGF) due to a decision of the German Parliament.
Furthermore, we would like to thank all industrial partners who support this research project.
Funded by
Network
Project Partners